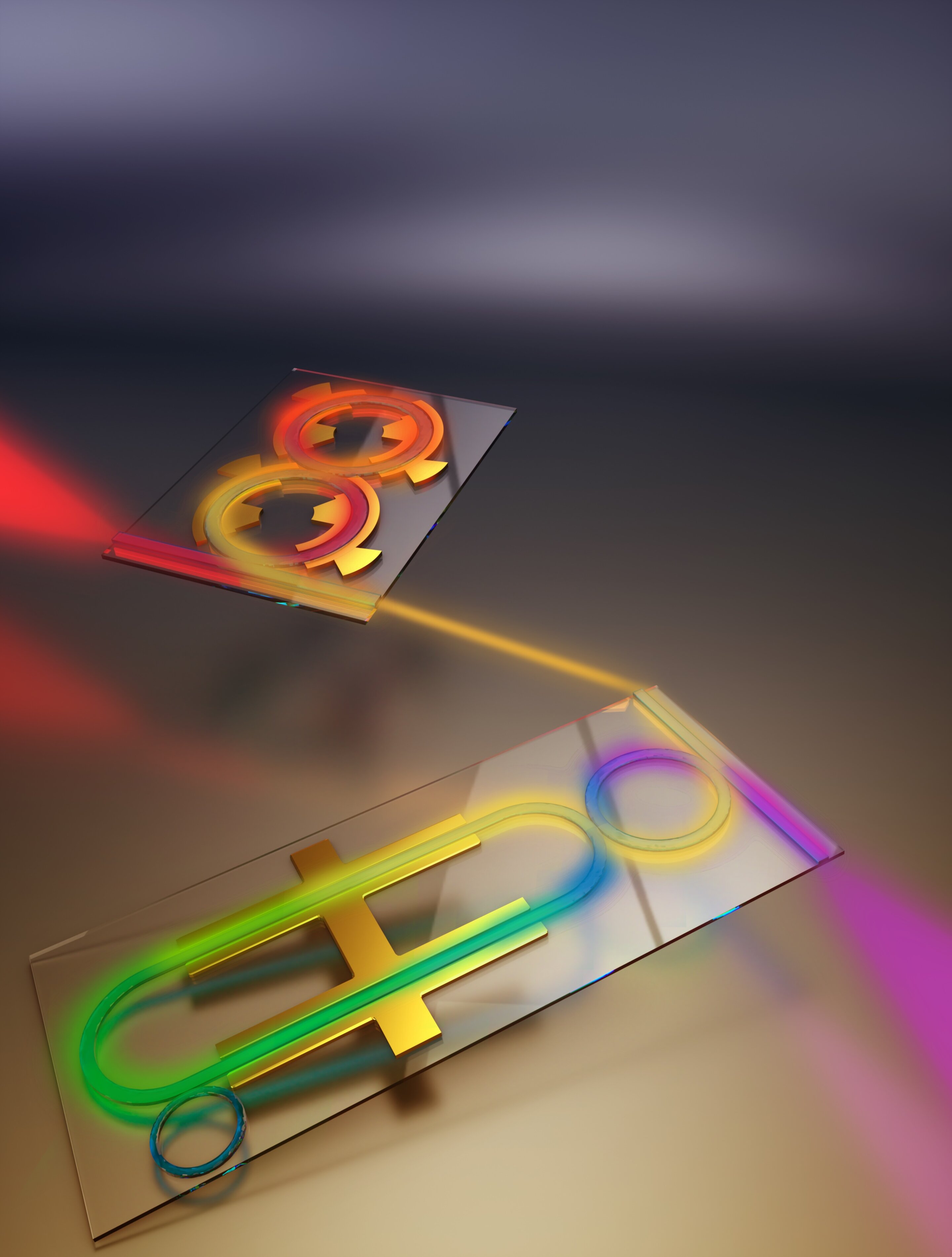
The top device has two coupled resonators. The input light travels through the resonators and enters as one color and the other as another. The bottom device has three coupled resonators: a small ring, a long oval, and a rectangular-shaped one. As light speeds around the racetrack, it cascades into higher and higher frequencies, causing a shift as high as 120 gigahertz. Second Bay Studios is a part of Harvard SEAS.
The ability to precisely control and change properties of a photon gave rise to a wide range of communication technologies, including the Internet. The properties of a photon will need to be more controlled in the next generation of technologies.
One of the hardest properties to change is a photon's color, otherwise known as its Frequency, because changing its energy is what it takes.
The most important frequencies for communications, computing, and other applications are found in the gigahertz range, which is where most frequencies are inefficient and can't convert light in the conversion process.
Researchers from the Harvard John A. Paulson School of Engineering and Applied Sciences have developed highly efficient on-chip frequencies that can convert light in the gigahertz range. Continuous and single-tone microwaves are used to control the frequencies.
Nature publishes the research.
The paper's senior author said that the frequencies could become a fundamental building block for high-speed, large-scale classical communication systems as well as emerging quantum computers.
The paper outlines two types of on-chip frequencies that can be used, one that can covert one color to another and the other that can cascade multiple shifts.
Lonar and his lab created the platform for each device.
It was long thought to be difficult to work with on small scales because of the efficiency of niobate. Lonar and his team demonstrated a technique to fabricate high- performance lithium niobate microstructures using standard plasma etching to physically sculpt microresonators in thin lithium niobate films.
Lonar and his team etched ring-resonators and waveguides on thin-film lithium niobate. The first device has a figure eight-like structure. The light travels through the resonators in a figure eight pattern, entering as one color and emerging as another. The device provides frequencies as high as 28 gigahertz. It can be configured to split a beam into two beams of different frequencies.
The second device has three coupled resonators, a small ring, a long oval, and a rectangular-shaped one. As light speeds around the racetrack, it cascades into higher and higher frequencies, causing a shift as high as 120 gigahertz.
The first author of the paper said that they were able to achieve this magnitude of frequency shift using only a single 30-gigahertz microwave signal. This is a new type of device. Attempts to shift frequencies larger than 100 gigahertz have been very difficult and expensive.
Lonar said that the work was made possible by previous developments in integrated niobate. The ability to process information in the frequency domain in an efficient, compact, andScalable fashion has the potential to significantly reduce the expense and resource requirements for large-scale photonic circuits.
Nature has more information about On-chip electro-optic frequencies and beam splitters. www.nature.com/articles/s41586-021-03999-x
Nature journal information.
Next generation quantum computers and networks could use on-chip frequencies in the gigahertz range.
The document is copyrighted. Any fair dealing for the purpose of private study or research cannot be reproduced without written permission. The content is not intended to be used for anything other than information purposes.