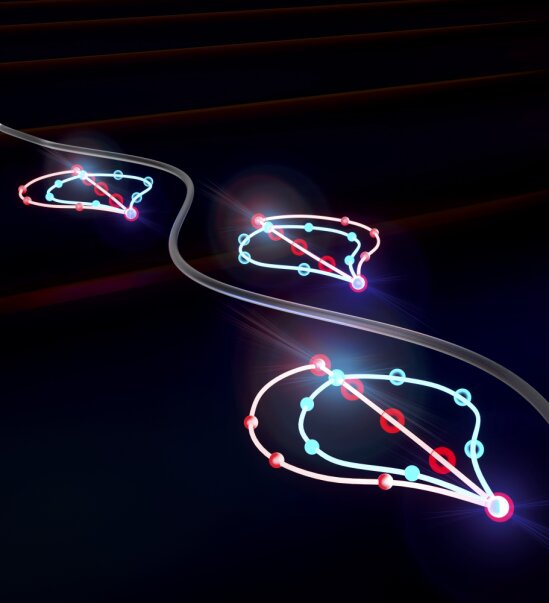
A near-IR laser, located in the lower right, separates two electrons (empty circle) from two types of holes (solid circle). The fluctuating electric field (gray wave) from the terahertz-laser accelerates the charges away from one another. The charges are pulled towards each other by the changing field. At that point, they combine to emit two flashes. The trajectories can be seen in one dimension, with time flowing from bottom left to top right. Credit: Brian Long
Lightspeed is the speed of light in the universe. Except when it's not. Anybody who has seen a prism turn white light into a rainbow knows how material properties can affect the behavior of quantum objects. In this instance, it is the speed at light propagates.
The behavior of electrons in materials is different from their behavior in free space. This makes it crucial for engineers and scientists who are interested in developing new technologies. "An electron's unique wave nature is extremely important. Co-author Joe Costello is a UC Santa Barbara graduate student studying condensed matter physics.
Co-lead authors Seamus O’Hara, Costello and Qile Wu, along with their collaborators, have developed a method to calculate the wave nature of this wave function, known as a Bloch wavefunction from physical measurements. Senior author Mark Sherwin from UC Santa Barbara, a professor in condensed matter physics, said that this is the first experimental reconstruction of a Bloch function. The findings of the team appear in Nature, more than 90 years since Felix Bloch described the behavior electrons in crystal solids.
As with all matter, electrons behave as waves and particles. Wavefunctions are mathematical objects that describe their wave-like properties. These functions can have real and imaginary components which makes them "complex" functions. The value of an electron’s Bloch wavefunction cannot be measured directly. However, it is possible to observe properties that are related to it.
Sherwin stated that understanding Bloch wavefunctions is essential to design the devices engineers envision for the future. O'Hara explained that electrons can get bumped around in materials, causing wavefunction scattering. This happens very quickly, in the order of 100 femtoseconds (less that one millionth one millionths of a second). Researchers have been unable to measure the electron's wavelike properties in the material, which has hindered their ability to reconstruct Bloch's wavefunction.
The Sherwin Group was the perfect group of people with the right equipment to take on this challenge.
To conduct their experiment, the researchers used gallium arsenide as a simple material. The material's electrons are stuck in bonds between Ga atoms and As atoms at the beginning. They excited the electrons using a low-intensity, high frequency laser. Some electrons are made more mobile by this extra energy. Every electron that is released leaves behind a positively charged hole, which acts a little like a bubble in water. Sherwin explained that there are two types of holes in gallium arsenide. They are "heavy" and "light" holes. These behave like particles with different masses. This subtle difference was crucial later.
A powerful terahertz-laser was creating an electric field that oscillates within the material, which could speed up these new unfettered charges. If the mobile electrons or holes were created at the correct time, they would move away from one another, slow down, stop, speed towards each other, and then recombine. They would then emit a pulse, known as a sideband with characteristic energy. Sideband emission encoded information about quantum wavefunctions, including their phases and how far apart they were from one another.
Mark Sherwin (bottom left) explains the workings of the free electron laser. The large yellow tank is used to accelerate electrons. They are directed along the beam line into the wigglers at far left. Credit: UC Santa Barbara
Their Bloch wavefunctions, which were accelerated at different rates by the terahertz light and heavy holes, acquired different quantum phases before recombined again with the electrons. Their wavefunctions interacted with one another to produce the final emission as measured by the apparatus. This interference also determined the polarization for the final sideband. It could be circular or rectangular even though both lasers had polarizations that were linear.
Qile Wu, a postdoctoral researcher, has figured out how to connect the quantum theory and the experimental data. Qile's theory only has one parameter: a real-valued number connecting the theory to the experiment data. Wu stated that there is a simple relation between the quantum mechanical theory and the real-world experiment.
Seamus O'Hara (a doctoral student in Sherwin's Sherwin group) said that Qile's parameter accurately describes the Bloch wavefunctions we create in gallium arsenide. This can be obtained by measuring sideband polarization, and then reconstructing the wavefunctions. These vary depending on the angle at the hole is propagating within the crystal. Qile's elegant theory links the parameterized Bloch wavesfunctions to the type light we should be studying experimentally.
Sherwin explained that Bloch wavefunctions are crucial because they can be used for nearly any calculation involving holes.
Scientists and engineers currently rely on theories that have many unknown parameters. Sherwin stated that if Bloch wavefunctions can be accurately reconstructed in a variety materials, then this will help to design and engineer all sorts of useful and fascinating things, such as lasers, detectors, and quantum computing architectures.
This accomplishment is the result over a decade's worth of hard work, a motivated team, and the right equipment. This research project was born out of a meeting between Sherwin Liu and Renbao Luu at the Chinese University of Hong Kong at a 2009 conference. He said that it wasn't like they set out to measure Bloch wavefunctions 10 years ago. "The possibility developed over the course of a decade."
Sherwin saw that the unique, small-sized UC Santa Barbara Free Electric Lasers could produce the powerful terahertz electrical fields needed to accelerate and collide electrons. They also possess a highly tuned frequency.
Although the team initially didn't understand their data, it was difficult to realize that sideband polarization was crucial to reconstructing wavefunctions. Sherwin said that they struggled for several years to understand the data. "With Qile's assistance, we finally figured out that the sideband polarization was telling us a lot."
The team has now validated the measurement and interpretation of Bloch wavefunctions using a familiar material. They are eager to expand their techniques to new materials and exotic quasiparticles. Costello stated that they hope to attract interest from groups working with new materials and interested in learning more about the Bloch Wavefunction.
Continue reading Physicists offer promising approaches to harnessing exotic electronic behaviour
J. B. Costello and al., Reconstructions of Bloch wavefunctions for holes in semiconductors, Nature (2021). Information from Nature J. B. Costello et al, Reconstruction of Bloch wavefunctions of holes in a semiconductor,(2021). DOI: 10.1038/s41586-021-03940-2