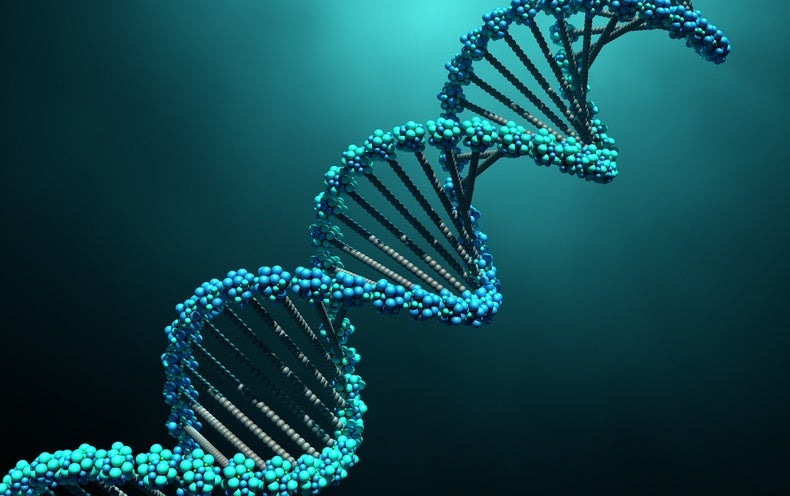
Gene therapy has been successful after many setbacks since the beginning of the 21st century. It is now being used to treat a variety of diseases, including neuromuscular disorders, cancer, and blindness. However, the success of gene therapy is not always easy to measure. Although some of these treatments have been successful in relieving disease, they come at a steep price and can be difficult to access. While other therapies may alleviate symptoms, they do not address the root cause.
Although it is possible to cure all patients, this is not possible in many cases, according to Julie Crudele, a neurologist and gene therapy researcher at University of Washington. Even small advances can lead to ongoing progress, she says, pointing out research with Duchenne muscular disorder patients: We learn important things in most clinical trials.
Gene therapy researchers now have a growing number of successful gene therapies to their credit thanks to this new knowledge and unwavering investigations. These are the four most promising.
Gene Swaps to Prevent Vision Loss
Many babies are born with severe visual impairment due to retinal diseases. This can lead to complete blindness. Some of these babies can now benefit from gene therapy developed by Jean Bennett and Albert Maguire who are now ophthalmologists at The University of Pennsylvania.
The pair had not yet identified any of the genes that could cause blindness or vision loss when they began their research on retinal disease in 1991. RPE65 was identified as a potential target gene by researchers in 1993. Bennett and Maguire developed a therapy that targeted this gene in three dogs suffering from severe vision loss seven years later. It restored vision for all three.
Leber congenital autism (LCA) is the best inherited condition in humans that corresponds to the dog's vision loss. LCA stops the retina, which is a layer of light sensitive cells at the back, from responding to light and sending signals to the brain. Uncontrolled shaking of the eyes (nystagmus) can lead to blindness. The condition is most commonly characterized by total blindness at age 40. Researchers have found that the disease is caused by mutations or deletions in any of 27 genes involved with retinal function and development. Gene therapy was the only cure.
Although mutations in RPE65 are only one cause of inherited redesitrophies, it was one that Bennett and Maguire were able to address. Researchers used an AAV (harmless adenovirus) to program the virus to locate retinal cells, insert a healthy copy of the gene, then injected the virus directly beneath the retina into the patient's eye. After a series clinical trials, voretigene-neparvovecrzyl was approved by the Food and Drug Administration in 2017. It is used to treat any heritable retinal disorder caused by mutations in the RPE65 gene. Luxturna is the FDA's first in-vivo gene therapy. This delivers the genetic material to the target cells within the body. Ex-vivo therapies delivered the genetic material to the target cells in body samples, which are then reinjected.
Spark Therapeutics, which makes Luxturna estimates that around 6,000 people in the world and between 1,000 to 2,000 in the U.S. might be eligible. It is also noteworthy that Luxturna was given orphan drug status by the FDA, which allows for the FDA to encourage the development of rare disease treatments. This didn't reduce the price. The cost of the therapy is approximately $425,000 per injection or almost $1 million for each eye. Maguire claims that despite the high cost, there has yet to be anyone in the U.S. denied access because they are unable to pay.
The results of treatment are remarkable: Many patients who had previously been unable to see clearly have their vision restored quickly. Many patients reported seeing stars for the first-time after receiving injections.
Although it is not clear how long the effects last, Luxturna patients who were treated in a phase III trial in 2017 had their better vision three years later. Bennett said that a five-year follow up with 29 patients showed similar results. This is currently under peer review. They can do things that they could never have imagined, are more independent, and enjoy life more.
To Fight Cancer, Train the Immune System
Gene therapy is also being used to combat cancer. Chimeric antigen receptor T cell therapy (CAR) is an approach that programs the immune system to target cancerous cells. Steven Rosenberg, the National Cancer Institute's chief surgeon, was instrumental in the development of the therapy. He published the first results of a study on lymphoma treatment using the therapy in 2010.
Rosenberg states that the patient was suffering from massive disease in his abdomen and underwent a complete regression. This regression has now been ongoing for 11 years.
CAR T cell therapy uses white blood cells (called T cells) as the first line defense against pathogens. This treatment uses patients' own T cells. These cells are genetically modified to make them more responsive to cancer cells. The modified T cells are then infused back into patients. They can now recognize and attack cancerous cell types, reproduce, and stay alert for new encounters.
Researchers at the University of Pennsylvania presented results in 2016 from a CAR-T cell treatment called tisagenlecleucel for acute lymphoblastic Leukemia (ALL), which is one of the most common childhood types of cancer. All patients have mutations in their bone marrow DNA that cause them to produce large amounts of lymphoblasts. These undeveloped white blood cells accumulate in the bloodstream. Adults with ALL have a low chance of being cured and less than half live for more than five years.
CAR T cells can be extremely effective when directed against ALL. One modified T cell can kill up to 100,000 lymphoblasts. The University of Pennsylvania study found that 29 of 52 ALL patients who were treated with tisagenlecleucel experienced sustained remission. The FDA approved the treatment (produced by Novartis under the name Kymriah), for treating ALL. It was also approved for use against diffuse large-cell lymphoma the following year. This one-time procedure can cost upwards of $475,000.
CAR T cell therapy can be dangerous. CAR T cell therapy can have severe side effects. This includes cytokine-release syndrome (CRS), which is a dangerous inflammatory response. It can range from mild flulike symptoms in less severe conditions to multiorgan failure or even death. CRS is not a condition that can be caused by CAR T therapy. It was first discovered by researchers in the 1990s, as an adverse effect of immunotherapies used in organ transplants. Doctors today have better understanding of how far they can push for treatment without creating CRS thanks to a combination vigilance and newer drugs. Rosenberg states that side effects can be dealt with immediately, which has resulted in a significant drop in serious illness and death due to cytokine release syndrome since the early days.
The remission rate for all patients who were treated with Kymriah in 2020 was around 85 percent. Over half of patients had no relapses within a year. Novartis will track the outcomes of all patients who have received the treatment for at least 15 years in order to better understand its effectiveness.
Precision Editing for Blood Disorders
A new player in gene therapy is being closely watched: in vivo gene edit using a system called CRISPR. This has been one of the most promising gene therapies ever since Jennifer Doudna & Emmanuelle Charpentier first discovered it in 2012. They shared the 2020 Nobel Prize in Chemistry for this feat. This June saw the publication of the first results of a small clinical trial that was aimed at treating sickle cells and closely related disorders, beta thalassemia.
Millions of people are affected by sickle cell disease. It causes crescent-shaped red cells to form that are more rigid and stickier than healthy cells. This can lead to anemia or other serious health problems. Beta thalassemia is another condition that affects millions. It occurs when someone's body produces less hemoglobin. This iron-rich protein allows red blood cells carry oxygen. If there are matching donors, bone marrow transplants might be a solution. However, other treatments for both conditions include blood transfusions as well as medications to manage the complications.
Both beta thalassemia and sickle cell disease are caused by single-gene mutations. These genes make them excellent candidates for gene-editing therapy. CRISPR–Cas9 uses DNA sequences taken from bacteria (clustered regularly-interspaced short palindromic repetitions or CRISPR) and an CRISPR–associated enzyme (Cas) to edit the patient's genome. CRISPR sequences can be transcribed onto RNA to identify and locate DNA sequences that are responsible for a specific condition. Cas9 is used to package the CRISPR sequences onto RNA that locates and identifies the target sequence. Cas9 then snips it from the DNA, thus repairing or deactivating any problematic genes.
Vertex Pharmaceuticals, CRISPR Therapeutics, and Vertex Pharmaceuticals presented unpublished results of a clinical trial with beta thalassemia patients and sickle cells treated with CTX001. This is a CRISPR/Cas9-based treatment. The therapy does not turn off a target gene, but instead boosts the production of healthy fetal hemoglobina genes normally shut down shortly after birth. CTX001 was used to treat beta thalassemia in fifteen people. After three months, all 15 patients had rapidly improved hemoglobin levels that no longer required blood transfusions. The same treatment was given to seven people suffering from severe sickle cell disease. All of them showed an increase in hemoglobin levels and reported no severe pain for at least three months. These improvements lasted for more than a year in five patients with beta thalassemia, and two with sickle cells. Patients are still being enrolled in the trial, which is currently ongoing. Vertex spokesperson said that it plans to enroll 45 patients and file for U.S. authorization as soon as 2022.
A Potentially Lethal Illness Can Be Derailled
Spinal muscular atrophy (SMA), a neurodegenerative disorder, is when motor neuronsthe nerves controlling muscle movement and connecting the spinal cord to organs and musclesdegrade, malfunction and eventually die. It is most commonly diagnosed in toddlers and infants. It is caused by a genetic mutation that prevents the production of a protein necessary for maintaining motor neurons.
SMA is categorized according to severity. It's based on how much motor neuron proteins a person's cells can produce. Even the most basic functions such as breathing and swallowing can be difficult in the most severe cases (type I). The 90 percent mortality rate for infants with type I SMA has been a historical record.
Adrian Krainer is a Cold Spring Harbor Laboratory biochemist. He first became interested in SMA in 1999 at a National Institutes of Health workshop. Krainer was studying how RNA mutations can cause cancer and other genetic diseases. Researchers suspected that there might be a defect in the splicing process, which could have been the source of SMA. Before RNA can be translated from the DNA template it must be edited or spliced to messenger RNA (mRNA). Some sequences are removed (introns), while others (exons), are added.
Krainer noticed similarities between SMA defects and the mechanism he was studying: a mistake that occurs during RNA splicing when an exon is accidentally lost. SMA patients were missing SMN1, a crucial sequence of gene sequences.
Krainer believes that if we could solve the problem of this exon being skipped, and could also find a way to fix it, this could be a great option for all [SMA] patients. Antisense therapy was the solution that he and his colleagues found. It uses single strands synthetic nucleotides to transmit genetic instructions directly to cells. [See The Gene Fix]. SMAs cases are different because the instructions inducing SMN2 (a motor neuron gene that normally produces small amounts) causes SMN1 to fail. SMN2 can then produce more motor neuron proteins and fill in for SMN1. In 2010, the FDA approved the first clinical trial of the method. The FDA also approved nusinersen, which is now known as Spinraza. The therapy cannot be integrated into the genome so it must be administered every 4 months to maintain protein production. It is also extremely expensive. A single Spinraza treatment can cost as high as $750,000 the first year, and as high as $375,000 each year thereafter.
It has been used to treat more than 10,000 people worldwide since 2016. Spinraza cannot restore motor function completely because one motor neuron gene doesn't have enough protein to do that. However, it can help children living with any of the four types SMA live longer, more active lives. Spinraza has been shown to improve patients' motor function. This allows them to stand, breathe, swallow, and even sit up on their own. Krainer states that patients receiving treatment very soon after birth are the most affected. This is because they have been diagnosed through newborn screening. This will allow you to prevent the onset of the disease for several years, and possibly forever.
This article is part Innovations In: Gene Therapy. It was created with financial support from Pfizer.