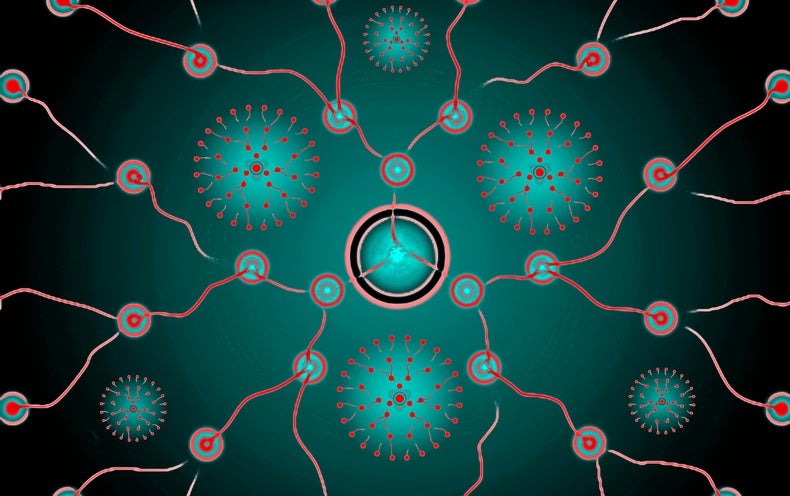
Recent papers highlight the growing interest in nanomaterials as antiviral countermeasures. Nanotechnology provides drug developers with a range of options that can be used in conjunction with antiviral drugs, as well as traditional small molecules and antibodies that prevent viral replication. These include viral-envelope inhibitors, cell-membrane decoys and virus binders. Some researchers believe that these materials will soon be available for clinical translation thanks to the funding boost from the COVID-19 pandemic.
SARS-CoV-2 has been defeated by nanomaterials. Moderna and PfizerBioNtech vaccines rely on lipid-nanoparticles to deliver mRNA into the cells. The promise of nanoparticles as small-molecule antiviral drug delivery systems is also being demonstrated, building upon decades of research in this area.
The urgency of the COVID-19 epidemic is driving interest in therapeutic nanomaterials. These materials can stop viruses from spreading and not just act as vaccine delivery vehicles. Joshua A. Jackman, South Korean University's Joshua A. Jackman, said that many of these nanomaterials can interact directly with virus particles, disrupting or binding to them.
Antiviral nanomaterials target the chemical and physical properties of many viruses, unlike traditional therapeutics that target one virus species. Recent papers describe antiviral strategies that use DNA-based nanostructures to trap virus or modify polymers to act as cell membrane decoys. Others break down viral membranes to prevent infection. These nanomaterials could be useful in countering pandemics because they can be quickly formulated and have activity against a wide range of viruses.
While much of this research is still restricted to academic labs and a few companies are working on antiviral nanomaterials, a lot of it is being done in the private sector. New opportunities are emerging due to the devastation caused by COVID-19 and the need to be prepared for possible future viral pandemics.
The Antiviral Program for Pandemics was launched by the Biden administration in June. It provides $3 billion for research to develop new antivirals capable of fighting SARS-CoV-2, and other pandemic viruses. According to Liangfang Zhang, University of California, San Diego, this new funding stream will stimulate and support further research and development in antiviral nanomaterials. COVID has changed the landscape and we now need more ready-made solutions to emerging viruses.
Many viruses depend on sugar-linked proteins (glycoproteins), which are found on their surfaces. Nanomaterials that mimic these attachment points could potentially be used as antivirals. Zhang is developing nanosponges to stop viruses. Zhang's team begins with human cells, such as red blood cells and immune cells called macrophages. They remove the contents of the cells to make the membrane. Then they cut the membrane into thousands or vesicles that measure approximately 100 nanometers in width. Then they add nanoparticles made from a biocompatible and biodegradable polymer, such as poly(lactic-co-glycolic acid). Each nanoparticle is coated with a cell membrane. This creates a stable core-shell structure which acts as a decoy for a human cell. To stop a virus from entering host cells, the nanosponges use binding points in their membranes.
These nanosponges are effective against a range of viruses and bacteria in vivo, and Zhangs San Diego-based spin-out company Cellics Therapeutics plans to begin a clinical trial next year of its lead candidate, a nanosponge carrying a red blood cell membrane that is effective against methicillin-resistant Staphylococcus aureus (MRSA) pneumonia. Cellics also uses macrophage membranes for similar antiviral nanosponges. Zhang says that there are many types of viruses and that each virus can have different variants. However, to infect humans, they must interact with their hosts cells via receptors.
Zhang discovered that cells coated with membranes made from human macrophages or human lung epithelial types II cells were able to trap SARS-2 and prevent infection in vitro. The membranes on these nanosponges sport the receptors angiotensin-converting enzyme 2 (ACE2) and CD147, to which SARS-CoV-2 binds during infection. Zhang's team has also published unpublished results of an in-vivo study on mice that showed efficacy against coronaviruses and no evidence for toxicity.
Starpharma, which is based in Abbotsford (Melbourne, Australia), mimics host cells to fight viruses. It produces synthetic polymers with a branched structure known as dendrimers. They measure approximately 3-4 nanometers in width. Each dendrimer's outer surface is covered with naphthalene diulfonate groups. This is similar to the heparan sulfate prooglycans that are found on host cell membranes. These molecules are where many viruses stick.
Starpharma has already products that use a dendrimer called SPL7013 to protect against bacteria and viruses. VivaGel is an example of a condom lubricant that uses SPL7013. Starpharma introduced Viraleze, an antiviral nasal spray that contains SPL7013. It is currently registered in Europe and India as a medical device. After the U.K.'s June referendum, Viraleze sales in the U.K. were stopped. Concerns were raised by the Medicines and Healthcare Products Regulatory Agency about marketing claims.
The company released research in August showing that Viraleze could prevent SARS-CoV-2 infections in mice. The nasal spray was administered before and after SARS-CoV-2 exposure. It reduced the viral load in the blood, lungs, and trachea of the animals by more than 99 per cent. According to the company, a clinical safety study that has not been peer-reviewed yet showed that Viraleze's dendrimer was not absorbed into the body and had no side effects.
Jackie Fairley, CEO of Starpharmas, believes that Starpharmas' dendrimer may prove useful in the future pandemics. She says it is a stable material that can be quickly formulated into a product and has activity against a wide range of viruses. The company is currently planning to conduct larger animal studies to confirm Viraleze's activity against SARS-CoV-2.
Antiviral nanomaterials can be precisely designed to trap viruses. Rainer Haag, a German researcher at the Free University of Berlin, is using silica nanoparticles to cover viruses with spikes between 5-10 nm tall. To enhance binding or to counterviral compounds like zanamivir, the spikes can be decorated using sialic acid sugars. We maximize the binding by matching the morphology and virus of the virus, Chuanxiong Nie, a Haags postdoc who has been leading this work, said. The particles were able to prevent the infection of cells with the influenza A virus in vitro. Now, the team hopes to develop spiky nanoparticles that are active against SARS-CoV-2. As part of an $1.8 million grant ($2.3 million), the Berlin University Alliance supports this work.
Another possibility is star-shaped DNA scaffolds. Xing Wang, University of Illinois at Urbana-Champaign, has created such structures that carry DNA aptamerssingle strandedDNA molecules capable of binding to antigens at multiple locations on dengue fever virus's surface. Because of its physical bulk and negative charge, the virus is prevented from attaching to host cells and shutting down infection. In vitro data from the team, which is currently being peer-reviewed, shows that certain DNA stars are capable of inhibiting SARS-CoV-2 infections. Wang hopes to make the DNA stars commercially through Atom Bioworks, a North Carolina spin-out.
Hendrik Dietz, Technical University of Munich, is currently working on a form of DNA origami. They have created shells from DNA large enough to swallow entire viruses. To hold on to trapped viruses, the interior of these self-assembling icosahedralshells can be lined using binders such as antibodies. Dietz believes that nanoshells may reduce viral load in acute infections.
Researchers created triangular DNA structures which can be assembled into shells of varying sizes and shapes, ranging from 90 to 300 nanometers in width. They created viruses-sized openings by altering the DNA sequences of the triangular building blocks. These shells were shown to be capable of binding viruses like adeno-associatedvirus serotype 2, and preventing them from infecting human cell. Christian Sigl, a graduate student in Dietzs' lab, said that the advantage of our shells was the ability to attach a variety of virus binders and switch them very easily. He says that the shells can be tailored to bind any virus. Dietz is the coordinator for Virofight, a 3.9 million project that was launched in June 2020 by the European Commission. It aims to create a shell that traps SARS-CoV-2 and then test it in mice.
Nanomaterials can do more than just binding viruses. They also disrupt the viral membrane to prevent infection. Although viral genomes are enclosed by a protein-based capid, in many cases (including that of SARS CoV-2), that capsid is protected by a phospholipid bilayer, which is crucial for virus attachment to cell membranes. This viral envelope, unlike bacterial membranes is acquired directly from the host cell membrane. Newly created virus particles are released into infected cells. Jackman states that this envelope is crucial for virus structure and infection. People don't realize that the lipid membrane can be druggable.
NanoViricides is a company based in Shelton Connecticut. It uses soluble polymer surfactants to disrupt viral membranes. These surfactants form micelles that are spherical. These nanoviricide structures can be decorated with up to 1,200 different ligands such as peptides that bind to viral Glycoproteins. The micelles, which are spherical aggregates that look like oil in water emulsions in a way, fuse with the virus membrane and infuse it with toxins, making it impossible to infect host cells.
Although the company was preparing for a clinical trial for a topical anti-viricide to treat shingles, it shifted its focus last year to COVID-19. It published positive results in vivo from two nanoviricides against SARS/CoV-2 in March. One of the nanoviricides contained the antiviral molecule Remdesivir as its core. Both nanoviricides had significantly longer survival times than remdesivir alone in rats with fatal coronavirus lung infections. The company said that although the study results are not yet peer reviewed, it is ready to start clinical trials with the nanoviricides.
Jackman is also working on antiviral proteins that will fit into the viral membrane. These peptides then aggregate to form pores. Jackman says that once a certain number of holes are formed in a membrane it collapses. He has successfully used this strategy to treat the Zika virus in mice.
It is still early days for many of these technologies. Although it is a niche market, I believe its growth is steady. There is certainly interest in it, according to Kathie SeleyRadtke, a medical chemist at University of Maryland, Baltimore County. She develops small-molecule antiviral drugs and is the president-elect of International Society for Antiviral Research. We can't ignore any possibility at this time, as COVID is so severe.
Jackman says that both pharma and biotech companies generally take a cautious approach when it comes to therapeutic nanomaterials. Concerns remain about the bioaccumulation of nanoparticles and long-term side effects. He says that recent developments with lipid nanoparticles for mRNA vaccines clearly show that nanomaterials are useful in fighting viruses. This may help to boost confidence.
Another problem is the diversity of protocols used in in vivo studies of these materials. This makes it difficult to compare. Some protocols require that the antiviral nanomaterial be preincubated with the virus before being administered to an animal. Others involve giving the antiviral before the animal is exposed to a virus. Jackman recommends that researchers agree on standard animal models and performance benchmarks to help more nanomaterials make it into clinical trials. Then, they should focus on the evaluation of antiviral nanomaterials in infected animals. Jackman states that material science is amazing for all of these concepts. This is where I believe the next frontier lies in translating it.
This article was published with permission on October 7, 2021.