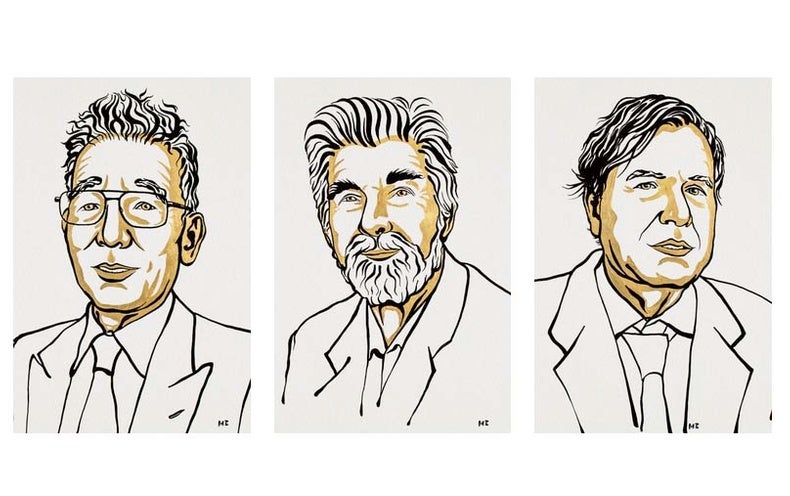
Complex systems are complex. This is a paradox that is often overlooked. Kunihiko Kaneko is a University of Tokyo physicist who says that complex systems are not easily defined. However, complex systems are composed of many interconnected elements that often exhibit chaotic or dynamic behavior.
For the first time, this year's Nobel Prize in Physics was specifically awarded to research in complex systems, including climate change. Half the prize was awarded to Syukuro Manabe, Princeton University, and Klaus Hasselmann, Max Planck Institute for Meteorology, Hamburg, Germany for their physical modeling of Earth's climate and reliable prediction of global warming. The second half went to Giorgio Parisi, Sapienza University in Rome for discovering the interplay between disorder and fluctuations at all scales of physical systems, from atomic to planetary.
The recognition was especially meaningful for those who work in neglected fields. Marc Mzard (physicist and head of the Cole Normale Suprieure France) said that he was touched by the recognition. He is also a Parisian colleague. Similar thoughts were shared by climate scientists about the award. It is, in fact, long overdue according to Shang-Ping Xie (an oceanographer at Scripps Institution of Oceanography, San Diego).
The Nobel Committee for Physics confused many observers by grouping seemingly unrelated research within the vague umbrella of complex system. This led to blurred headlines like Physics Nobel Rewards Work on Climate Change, Other Forces. However, a closer look reveals connections about the goals of science and how scientists can solve seemingly impossible problems.
In the 19th century and most of the 20th, physicists developed a greater understanding of complex microscopic and macroscopical systems that are full of random motions and disorder. Many of the tools they created (many of them still relied on by Parisi, Hasselmann and Parisi) were useful in many areas. They could calculate how much sun energy Earth absorbs, describe the movement of pollen grains on water, or explore the magnetic properties theoretical materials.
This connection has also a philosophical component. The Nobel Committee for Physics concluded its paper about the scientific background for the prize in physics 2021 at the end.
We cannot fully understand any system's behavior if we don't examine its origins. Only after considering these origins can we see that global warming is real, and is due to human activities. We also understand that many of the phenomena observed in nature are a result of an underlying disorder. It is important that we embrace uncertainty and the noise that surrounds us, as this is the first step towards predictability.
If this is still not satisfying, it's worth remembering that the committee is a complicated system full of uncertainty, disorder, and complexity.
Broken Glass
In the 1920s, quantum mechanics was just beginning. Physicists created a simple model that described magnets such as the ones found on fridges. This Ising model describes magnets as a lattice made of atoms. Each atom acts as a tiny magnet, with each atom having a direction that is either upward or downward. A ferromagnet is formed when all the atomic magnetic magnets align in one direction. They are antiferromagnets if they move in opposite directions.
Nature had more to offer than two types of magnetism. After several metal alloys had strange magnetic behaviors, Philip Anderson and Sam Edwards, both late theorists, proposed a new type of magnet that included atomic magnets in some pairs, while others were randomly anti-aligned. The new magnet was called a spin-glass because of its disordered magnetic orientation. This is similar to what happened in glass crystals.
Imagine a trio of atomic magnets in triangle. If the conditions are met, then two magnets can be used to fulfill it. The third magnet is left in limbo. The impossible situation made spin glass unworkable. There was no clear way to order it. Inherent disorder can manifest in infinite numbers of unpredictable states. Therefore, physicists used spin glass to calculate its properties by averaged many copies of the system. This is known as the replica trick.
According to Daniel Stein, a New York University physicist, the result violated several thermodynamic principles. It was obvious that this was not the right solution. Their symmetry or similarity was broken.
Parisi achieved replica symmetry breaking in 1979. Stein uses this physical example to illustrate the point: Imagine a protein strand embedded in a solution. The temperature drops and the same protein can freeze, forming many different ground states or configurations. Parisis calculations were able to account for the innumerable ground states of spinglass, which is strange.
Stein said that people became very excited about it. This solution has the potential to solve the problem of disordered system? Many other researchers from different disciplines, including neuroscience, evolutionary biology, and computer science found Parisis' solution fascinating because it offered a novel, rigorous way of thinking about the various configurations of disordered system. It gave new perspective to optimization questions such as the traveling salesman question and the science behind neural connections.
This is an example of order and disorder. The Spin glass is about as random as it gets. Stein says that this order is a sort of order that no one would have predicted. Although the ground states may be different in their nature, they are connected because they all derive from the same higher energy state.
Parisi didn't close the book on spin-glass. Many questions remain about its properties, including how replica symmetry breaking works beyond idealized equations.
Climate Chaos
The world we live in is chaotic and not disordered. Even small changes in the initial conditions can have huge effects on systems like the weather. The famous butterfly effect is a reference to the aerodynamic influence of the flaps of wings of butterflies in Africa. This can affect the formation of hurricanes in the Atlantic.
Tony Broccoli of Rutgers University, an atmospheric scientist, said that Manabe was originally trained as a meteorologist. Manabe was thinking about complex systems and had to simplify them into something that the computers could understand.
Richard Wetherald and Manabe published the first computer model that simulated climate sensitivity to fluctuations in atmospheric carbon dioxide levels, which is the major culprit for human-made global warming. They simulated the climate using a single column and examined how convection relates to temperature variations.
Broccoli states that you can get many misleading results just by looking at the energy balance on the Earth's surface. Broccoli says that it was crucial to consider the whole air column when calculating the global temperature increase. Manabe and Wetherald had predicted that an increase of 2.4 degrees Celsius in the atmosphere's carbon dioxide concentration would cause an increase in global temperatures. Although it was a simple model with no feedback mechanisms for clouds, the answer was very similar to modern predictions using far more sophisticated methods.
Manabe created the first computerized global climate model for Earth in 1995. This model has many applications beyond carbon dioxide sensitivity and can be used to predict phenomena like El Nio.
While Manabe tried to reduce the impact of noisy weather on climate models, Hasselmann brought the noise to the forefront. Robert Brown (1827-1899), a 19-year-old Scottish botanist, was a major influence on Hasselmann's work. He described the bizarre dance of pollen grains within a quiescent droplet of water, which could be viewed through a microscope. Albert Einstein provided a mechanism to explain this Brownian motion eight decades later: despite the water's apparent stillness, the grains moved due to innumerable small, random collisions of atoms and molecules.
Hasselmann wondered whether the climate was something like those pollen grains or if the weather was like the endlessly restless atoms. If true, then climate was subject to internal variability due to random weather. This is independent from any external force, such as the sun's warming rays. Hasselmann proved that climate responds to random variability in 1976. His work was crucial because it took into account natural climate variability and helped climate scientists to determine how much warming was anthropogenic.
Jin-Song von Storch is a climate scientist at Hamburg's Max-Planck Institute for Meteorology.
This internal variability can have a large impact on the results. Xie estimates, however, that calculations of global warming could be off by up to 25% in certain cases.
Alfred Nobels will state that his prizes should be given to those who have provided the greatest benefit for humanity.
Another possibility is suggested by this years announcement. Xie states that physics applied to the greatest benefit of humanity is fundamental.