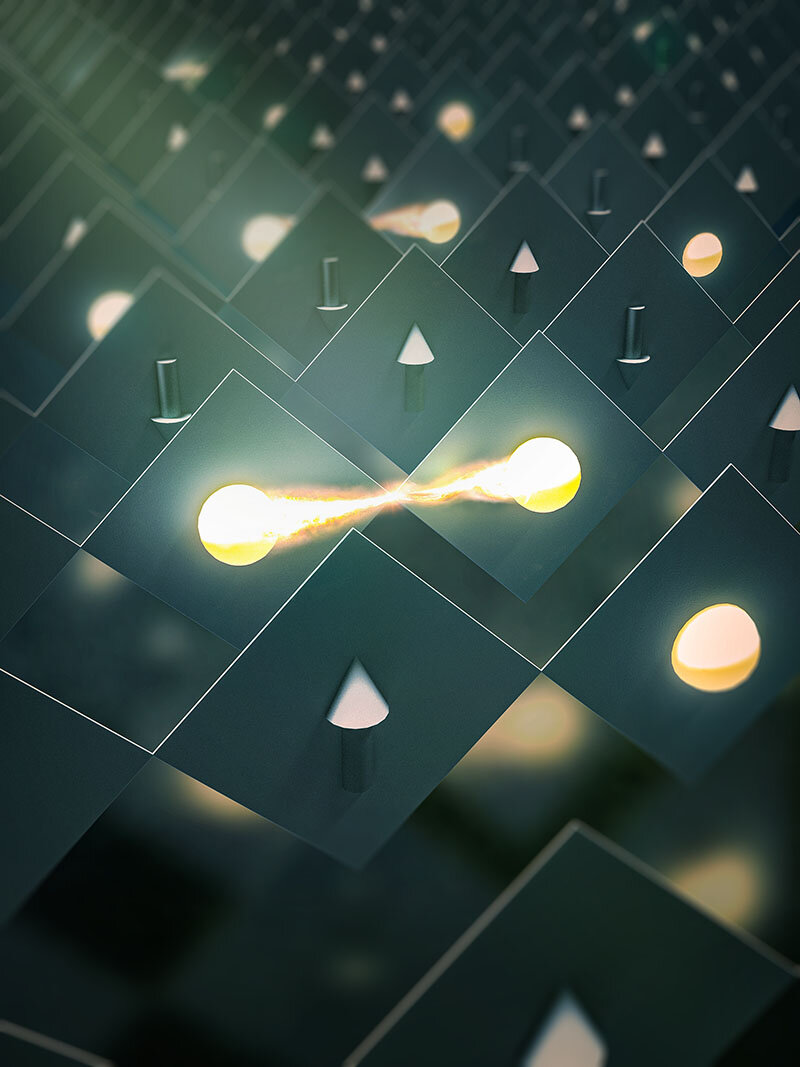
Illustration of an unusually strong attraction between electrons at neighboring lattice locations within a 1D copper oxide chain, or cuprate material. This is a material that conducts electricity with little loss at high temperatures. Clemson, SLAC, and Stanford researchers discovered an unusually strong near-neighbor attraction in a cuprate 1D chain. The doped material had been altered to increase its density. The unexpected strength of these attractions could be due to interactions with natural vibrations within the materials atomic lattice. This may have a role in cuprate supraconductivity. Credit: SCI–HUA
When scientists study unconventional superconductorscomplex materials that conduct electricity with zero loss at relatively high temperaturesthey often rely on simplified models to get an understanding of what's going on.
These quantum materials are known to be made from electrons which combine forces to create a kind of electron soup. Researchers have discovered this. This complex process would require far more computing power and time than anyone could imagine. So for understanding one key class of unconventional superconductorscopper oxides, or cupratesresearchers created, for simplicity, a theoretical model in which the material exists in just one dimension, as a string of atoms. These one-dimensional cuprates were created in the laboratory and their behavior was very consistent with the theory.
These 1D atomic chains were missing one thing. They couldn't be doped. This is a process in which some atoms are swapped for others to alter the number of electrons free to move about. Doping is one factor scientists can control to alter the behavior of materials such as these and it's crucial to making them superconducting.
A team of scientists from the Department of Energy's SLAC National Accelerator Laboratory, Stanford University and Clemson Universities has now created the first doped 1D cuprate material. The doped material was analysed by the scientists at Stanford and Clemson universities. They found that the most popular model for how cuprates can achieve superconductivity does not include a key ingredient. This is the unexpectedly strong attraction of neighboring electrons within the material's atomic structure or lattice. They suggested that this attraction could be due to interactions with natural lattice vibrations.
Today, the team published their findings in Science.
Zhi-Xun Shen is a Stanford professor and researcher with the Stanford Institute for Materials and Energy Sciences at SLAC.
He said that "now that we have done it", "our experiments show our current model misses an extremely important phenomenon that is present in the real material."
A picture of 1D copper oxide chains, or cuprate, that have been doped in order to release some electrons. This was part of a study by researchers from SLAC National Accelerator Laboratory, Stanford University, and Clemson Universities. Copper atoms are dark and oxygen atoms violet. Red springs are natural vibrations that vibrate the atomic lattice. This may cause an unexpectedly strong attraction (not seen) between neighboring electrons. Unconventional superconductivity, which is the ability to conduct electricity at high temperatures with little loss, may be due to this near-neighbor attraction. Credit: Greg Stewart/SLAC National Accelerator Laboratory
Zhuoyu Chen (a postdoctoral researcher at Shen's laboratory) led the experimental portion of the study. He explained that the research was possible because of a system developed by the team for creating 1D chains embedded into a 3D material, and then moving them into a chamber at SLAC’s Stanford Synchrotron Radiation Lightsource, (SSRL), for analysis using a powerful Xray beam.
He said that it was a unique set-up and essential for getting the high-quality data needed to see these subtle effects.
In theory, there are many ways to go from grids to chains
Hubbard is the most popular model to simulate complex materials. It is 2D and is based upon a grid that contains the simplest possible molecules.
Thomas Devereaux, a SLAC professor and Stanford SIMES investigator, supervised the theoretical portion of this research. It is not possible to verify that the mathematical calculations of the material's physical characteristics are correct. If they do not match experimental results, it is impossible to determine whether the calculations or theoretical model were wrong.
Scientists have used the Hubbard model for 1D chains of the simplest cuprate lattice, a string of copper atoms and oxygen atoms, to solve this problem. The 1D version can accurately capture and calculate the collective behavior electrons in materials made from undoped 1D chain. However, it has been impossible to verify the accuracy of the predictions for doped chains. This is despite having tried numerous times over the past two decades.
Chen stated that "Our greatest achievement was in synthesizing these didped chains." We were able dope them across a wide range of temperatures and obtain systematic data to pinpoint what we were seeing.
Clemson, Stanford, and SLAC used a technique known as angle-resolved fotoemission spectroscopy, (ARPES) to eject electrons in doped 1D copperoxide chains and measure their energy and direction. They were able to get a clear and precise picture of the behavior of the electrons within the material. This work was carried out at SLACs Stanford Synchrotron Radiation Lightsource. Credit: Zhuoyu Ch/Stanford University
One atomic layer at time
Chen and his coworkers sprayed a thin film of a cuprate substance called barium strontium-copper oxide (BSCO) onto a support surface within a chamber located at the specially designed SSRL beamline. The film's lattices and the surface were aligned in such a way as to create 1D chains of copper, oxygen and other elements in the 3D BSCO material.
Chen stated that they didped the chains by heating them with ozone and heat. This added oxygen atoms into their atomic lattices. Each oxygen atom pulls an electron from the chain. The freed electrons then become more mobile. These millions of free-flowing electrons can form the collective state that is the basis for superconductivity.
The researchers then moved their chains to another section of the beamline, where they were analyzed with angle-resolved photosemission spectroscopy (or ARPES). The researchers ejected electrons and measured their energy from the chains, giving them a clear picture of the behavior of the electrons.
Surprisingly strong attraction
The analysis revealed that electrons attracted to each other in doped 1D materials is 10 times stronger than predicted by the Hubbard model, according to Yao Wang, a Clemson University assistant professor who was involved in the theory part of the study.
Researchers suggested that the high level of "nearest neighbor" attraction could be due to interactions with phononsnatural vibrations, which jiggle the atomic structurework. Although conventional superconductivity is well-known, phonons have been implicated in some form of unconventional superconductivity. However, there are also indications that phonons could play an additional role in superconductivity occurring at higher temperatures in materials such as cuprates. This has not been proven.
Scientists believe that the strong near-neighbor attraction between electrons is present in all cuprates. This could be used to understand superconductivity in 2D Hubbard models and their kin. Scientists will have a better understanding of these puzzling materials.
Explore more A strong kinship is discovered between nickelate magnetism and cuprate superconductors in the first study of their magnetic properties
Further information: Science, (2021) Anomalously strong near neighbor attraction in doped 1D-cuprate chains Information from Science Journal: Science Anomalously strong near neighbor attraction in doped 1-D cuprate chains (2021). www.science.org/doi/10.1126/science.abf5174