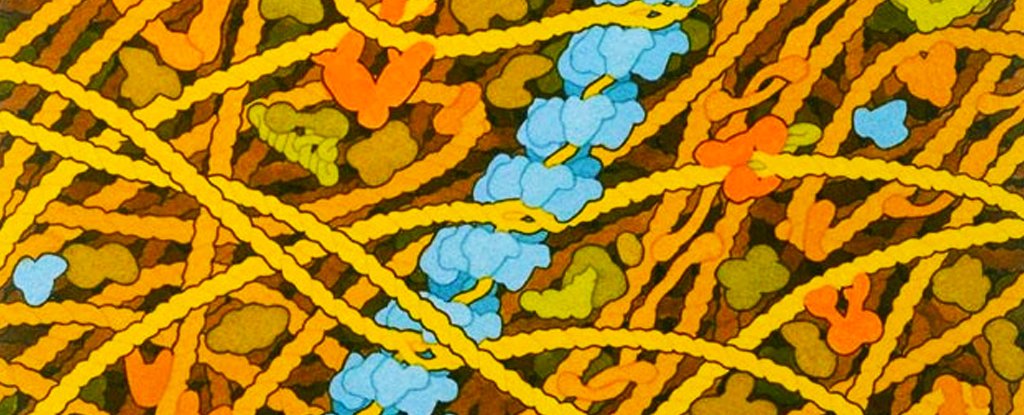
Most organisms can make the difference between life and death by fixing genetic breaks quickly and perfectly. Even the simplest changes to a sequence can lead to catastrophe, especially if they are responsible for a crucial function.
Biologists have spent the last half century studying the mechanisms that underlie DNA repair. One aspect of this process is still a mystery.
Researchers from Uppsala University, Sweden, have added details to how bacteria finds the templates that they need to repair genetic defects. They did this by marking DNA and enzymes with fluorescent tags and then watching the process unfold in real time in an Escherichiacoli model.
Homologous recombination is a method that most living organisms use to maintain their code order. It involves comparing two different versions of the same script to ensure no errors have been introduced.
A cell can check that the sequence was not damaged by holding it up next to a repair job.
RecA, a recombinase-protein RecA, is a key component of this process. This has been known by molecular biologists for some time. RecA is so important in maintaining DNA integrity that it's been found in almost every species.
A complex of proteins grabs hold of the broken ends of a DNA double-stranded ladder and trims it so RecA can do its job.
This is when the protein extends into a long cluster and forms a filament of nucleic acids and protein that can hold onto both the broken DNA strand and another intact ladder of unbroken genetic material.
Scientists know this. The filament must then find the correct sequence to be used as a comparison. It has been difficult to understand how the filament does this in such a short time. This is compounded by the many base pairs that must be checked among the complicated twists and turns of a chromosome.
Researchers grew thousands upon thousands of E.coli cells in tiny channels to better understand how the enzyme works. This allowed them to track individual bacteria as they were being experimented on.
Once the cells were in place, scientists used CRISPR gene editing to make precise breaks in the DNA. The scientists then labeled the ends with fluorescent markers to locate the break under a microscope.
Jakub Wiktor, Uppsala University molecular biologist, says that the microfluidic culture chips allow us to track the fate of thousands upon thousands of bacteria at once and to monitor CRISPR-induced DNA changes in real time.
Finally, they used antibodies as a way to locate RecA filaments. Then they went about their library searches.
The team was notified by a chemical alert when the repair was complete. The entire repair process took approximately 15 minutes on average.
Surprisingly it took only nine minutes for the protein's correct template to be found.
RecA's nucleoprotein fibril is the key to its success. This thread runs across the cell, grasping the chromosome and then sliding down to find a match.
Although it may not sound very efficient, this is really just like walking through a library looking for a book matching the call number.
Arvid Gynn says, "Since DNA ends are incorporated in this fiber, it suffices that any part of filament finds the precious templates, and so the search is theoretically reduced to three to two dimensions."
"Our model suggests this is the key for homology repair that's quick and efficient."
Although this research was done on bacteria, RecA's similarity throughout the biosphere makes RecA relevant for our bodies.
We now know the basics of DNA repair and can look out for potential problems. This will help us to understand the causes of cancer.
The research was published by Nature.