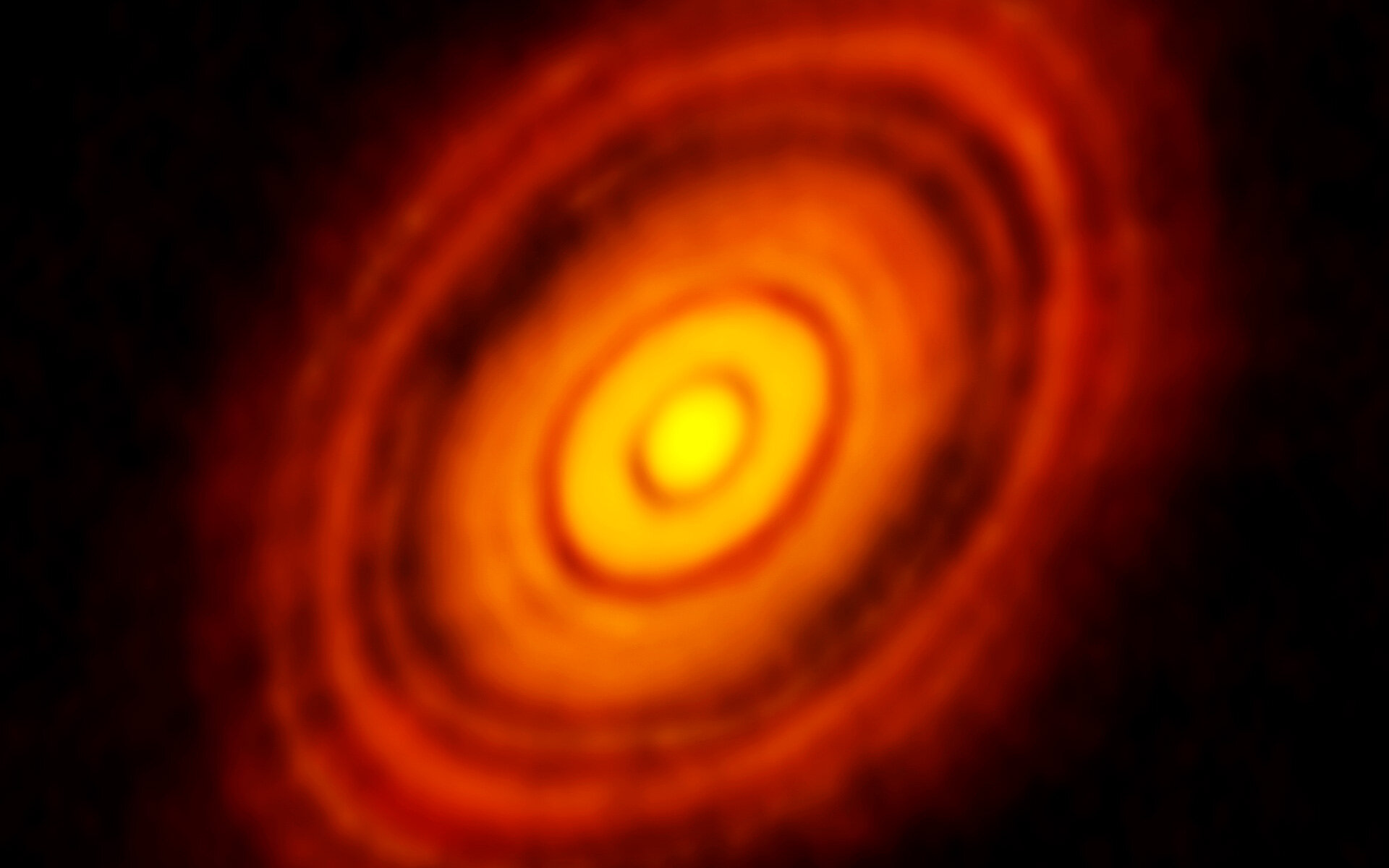
This is the most sharp image ever taken by ALMA, which is sharper than the NASA/ESA Hubble Space Telescope. It shows a disc around a young star. The new ALMA observations show the substructures of the disc that have never been seen before and show the possible positions of planets in the dark patches. ALMA (ESO/NAOJ/NRAO)
Astronomers have been able to link the properties of the inner planets of our solar system with the history of our universe, because of the emergence of ring structures in the swirling disk of gas and dust in which these planets were formed. The transition from an outer region where ice can form where water can only exist as water vapor is one of the basic physical properties associated with the rings. The simulation was used to explore different possibilities of inner planet evolution. Our solar system's inner regions are very rare and could be an outcome of that evolution. Nature Astronomy published the results.
For decades, the picture of planet formation around stars has remained the same. The search for explanations is an important part of current research. The inner planets in our solar system have properties that we can observe, thanks to an explanation found by a group of astronomy professors.
A swirling disk and rings.
The broad-stroke picture in question is as follows: Around a young star, a "protoplanetary disk" of gas and dust forms, and inside that disk grow ever-larger small bodies, eventually reaching a diameter of thousands of kilometers. Thanks to modern observational methods, the modern picture of planet formation has been refined and changed in very specific directions.
The first image taken by the ALMA observation was the most striking change. The image showed a disk around a young star with a nested structure of rings and gaps.
As the researchers involved in simulating protoplanetary disk structures took in these new observations, it became clear that such rings and gaps are often associated with pressure bumps. Changes in the size of dust grains are associated with those local changes.
There are three key transitions that produce three rings.
There are pressure bumps associated with transitions in the disk that can be linked to fundamental physics. It is too hot for silicate compounds to exist in any other state, because they are very close to the star. That means that planets can't form in that hot region. Any silicate gases that are below that temperature transition to a solid state. The pressurebump defines the inner border of planet formation.
The water snowline is a transition between water ice on the one hand and water vapor on the other. The lower pressure in Earth's atmosphere is the reason the temperature is so much lower than the standard 0 degrees Celsius. The CO snowline is at a lower temperature of 30Kelvin, which is the temperature at which carbon monoxide forms a solid ice.
pebble traps pressure bumps
What does this mean for planetary systems? The formation of planetesimals, the small objects between 10 and 100 kilometers in diameter, that are believed to be the building blocks for planets, can be aided by pressure bumps. The formation process starts with dust grains. Dust grains of a certain size tend to collect in the low-pressure region of a pressurebump, until they are stopped by the higher pressure at the inner boundary of thebump.
It becomes easier for those grains to aggregate into larger objects when the ratio of solid material to gas increases. Astronomers call the solid aggregate pebbles, they are between a few millimeters and a few centimeters in size.
Pressure bumps are part of the solar system.
The role of those sub-structures in the overall shape of planetary systems, like our own Solar system, was still an open question. This is the question that was asked by the group of people. They combined several simulations to find answers.
The astronomer created a model of a gas disk with three pressure bumps at the silicates-become-gaseous boundary and the water and CO snow lines. The growth of planetesimals and the formation of planetary embryos were simulations of how dust grains grow and fragment in the gas disk.
The asteroid belt between Mars and Jupiter is home to hundreds of smaller bodies, which are believed to be remnants or collision fragments of planetesimals that never grew to form planets.
There are variations on a planetary theme.
If the initial setup were just a little bit different, would the end result still be the same? Understanding which of the ingredients are the key to the outcome of the simulation is important. Bitsch and his colleagues analyzed a number of different scenarios with different properties for the composition and the temperature profile of the disk. The silicate and water ice pressure bumps are only in some of the simulations.
The results show a correlation between the appearance of our solar system and the ring structure of its disk. It was a complete surprise to me that our models were able to capture the development of a planetary system like our own.
As expected, planetesimals in those simulations formed naturally near the pressure bumps, as a "cosmic traffic jam" for pebbles drifting inwards, which would be stopped by the higher pressure at the inner boundary of the pressure bumps.
Our (inner) solar system has a recipe.
The researchers identified the right conditions for the formation of our own solar system if the region outside the innermost silicate pressure bump contains 2.5 Earth mass' worth of planetesimals.
A more massive disk would lead to the formation of "super-Earths" that are considerably more massive rocky planets. The super-Earths would be in close proximity of the host star. The boundary can explain why Mercury is not closer to the sun than any other planet.
Earth and Venus collect most of the material that will form their bulk from regions closer to the sun than the Earth's current path, according to simulations. The Mars-analogs in the simulations were mostly built from regions farther away from the sun.
How to build a belt of asteroids.
The simulations yielded a region that started out as sparse and empty of planetesimals, the beginning of the present-day asteroid belt of our solar systems. Some planetesimals from the zones inside of or directly beyond would stray into the asteroid belt region and become trapped.
As the planetesimals collided, the resulting smaller pieces formed asteroids. The simulations are able to explain the different asteroid populations, which are called S-types asteroids and C-types asteroids, which are mostly made of Carbon.
There are planets and a belt of planets.
The simulations show the beginning of the formation of giant planets just outside of the inner limit of water ice.
The most massive planetesimals would quickly gather more mass. The simulations didn't follow up on the evolution of those giant planets, which involved a tight group from which Uranus and Neptune migrated to their present positions.
The simulations can explain the final class of objects, which formed outside the outer pressurebump, which marks the inner boundary for carbon monoxide ice. It can explain the slight differences in composition between known Kuiper-belt objects, as the difference between planetesimals that formed originally outside the CO snowline pressurebump and stayed there, and planetesimals that deviated from the adjacent inner region of the giant planets.
Our rare solar system and two basic outcomes.
The spread of simulations led to two outcomes, one of which was that the inner and outer regions of the planetary system went their separate ways very early on. The formation of low-mass planets in the inner parts of the system is similar to what happened in our own solar system.
More mass can drift into the inner region if the water-ice pressure bump forms later than that. Evidence from the observations of those exoplanetary systems shows that the case is more likely than not.
Outlook.
The focus of the research was on the inner solar system and the planets. They want to run simulations that include the outer regions. The goal is to arrive at a complete explanation for the properties of ours and other solar systems.
The boundary between frozen water and water vapor and its associated pressurebump in the swirling disk of gas and dust that surrounds the young sun can be traced to some basic physics.
The results were published in the journal Nature Astronomy.
Nature Astronomy journal has information.
The properties of Mercury, Venus, Earth and Mars can be explained by Cosmic History.
The document is copyrighted. Any fair dealing for the purpose of private study or research cannot be reproduced without written permission. The content is not intended to be used for anything other than information purposes.